In a January 2023 publication by nuclear theory postdoctoral fellow Jennifer Rittenhouse West, a quantum chromodynamics (QCD) bond between quarks from different nucleons is proposed as the cause of short-range correlated pairs in nuclei – a fundamental particle physics explanation for roughly 20% of nuclear structure.
Nuclei were found to have their own internal structure composed of neutrons and protons arranged in shells of quantized energy by Nobel Laureate Maria Goeppert Mayer in 1948. Decades later it was shown, surprisingly, that not all nucleons are contained within shells. Nearly 20% of nucleons are paired into highly overlapped states called short-range correlations (SRC) and the cause of this pairing is unknown.
The uncertainty principle of quantum physics allows nucleons to suddenly come very close together in the nucleus – forcing their wavefunctions to strongly overlap – but the overlap lasts only momentarily before the nucleons must fly apart again. How the nucleon pairs in SRC stick together is a longstanding mystery. This is where the new work by Rittenhouse West comes into play.
At a fundamental particle physics level, QCD describes interactions between quarks, antiquarks and gluons, all of which have “color charge,” in analogy to the electric charge of quantum electrodynamics (QED). Two color-charged quarks can bind together into a diquark if they come into very close range, a process that typically only occurs within the close quarters of a single nucleon; for example, an up quark and a down quark inside a proton forming a diquark. However, the large nucleon-nucleon overlap due to the quantum uncertainty principle allows quarks from different nucleons to come close enough to form a diquark (see Fig.1 for a plot of the large wavefunction overlap between SRC nucleons in the nucleus). It is this attractive short-range diquark bond across a nucleon pair that is proposed to cause the correlation between nucleons. Rittenhouse West’s calculation of the diquark binding energy shows that certain diquarks – those made of up and down quarks whose spins cancel out – are energetically favored to form in the nuclear environment. In her model, the energy “borrowed” by the quantum uncertainty principle in order to force nucleons very close together is, at least temporarily, paid back in the form of significant diquark binding energy (see Fig.2 for a schematic of the diquark bond process).
Diquark formation between nucleons is poised to be a major leap forward in understanding the structure of nuclei in terms of the underlying particle theory of QCD if proven to be correct. Recent experimental results acquired at Jefferson Lab by a collaboration led by NSD scientists including Shujie Li and John Arrington are favorable to Rittenhouse West’s model.
[1] Jennifer Rittenhouse West, Nuclear Physics A 1029 (2023)
Diquark induced short-range nucleon-nucleon correlations & the EMC effect
[2] S. Li, R. Cruz-Torres, et al. with J. Arrington, Nature 609 (2022)
Revealing the short-range structure of the mirror nuclei 3H and 3He
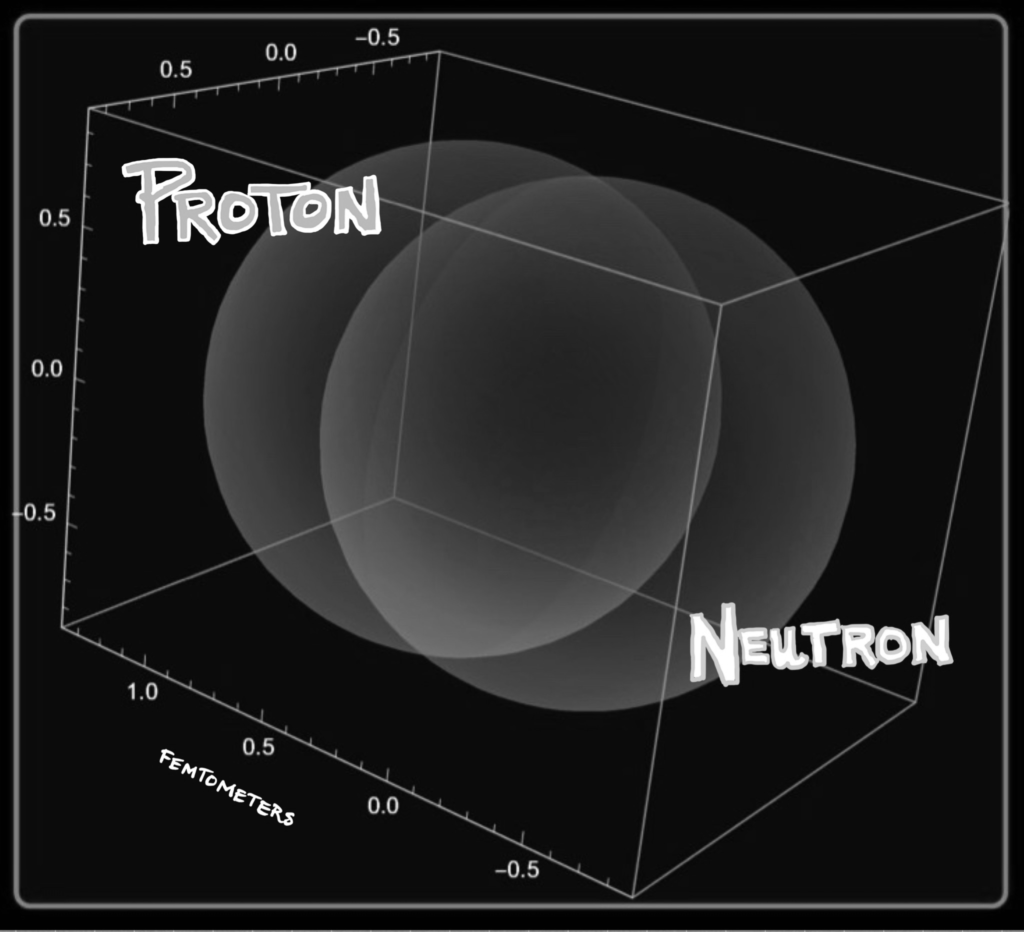
Figure 1: The overlap of two nucleons in a SRC. The relative momentum between them is 400 MeV/c (taken from neutron-proton SRC measurements in carbon-12), corresponding to a separation distance of ~0.5 femtometers – a very small separation considering the rms radius of a nucleon is 0.84 femtometers. Nearly 60% of the volume of each nucleon is within the overlap region. Fundamental QCD effects – like attractive potentials between quarks – are nearly certain to have an effect with such large wavefunction overlap.
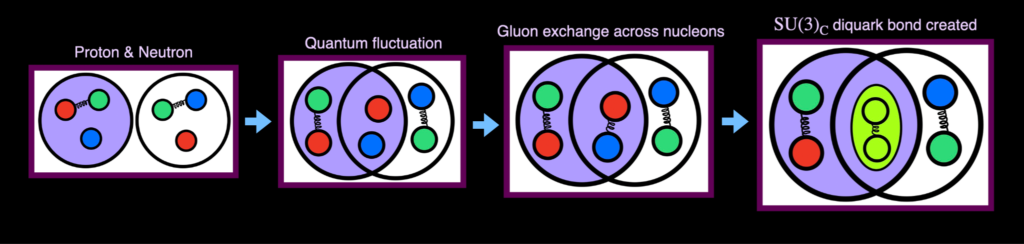
Figure 2: A diquark bond formed across a nucleon pair. Quarks are represented by “color-charged” circles in red, green and blue. Gluons, the mediators of the strong force, are represented by black spirals. The panels evolve from left to right as the nucleons begin as neighbors, undergo a quantum fluctuation into an overlapped pair, followed by gluon exchange between a blue quark from the proton and a red quark from the neutron, ending with the diquark bond formed as represented by the lime green oval in the final panel. The mathematical transformation of 2 quarks into a diquark changes the color-charge of the final state. The diquark is no longer a simple red quark-blue quark object, but an anti-green object, by the mathematical rules of the SU(3) group theory that QCD is based on. (Lime green was chosen to represent the anti-color charge called anti-green.)